Biomechanics of blood circulation (from wikiscripta)
Biomechanics of blood circulation describes the structure and mechanical properties of circulation, and thus explains how blood actually flows in the body.
Heart[edit | edit source]
See the Heart page for more details .
The heart is a hollow organ of the vascular system that propels blood through the bloodstream through rhythmic contractions . This function is made possible by the heart muscle - the myocardium . [1].
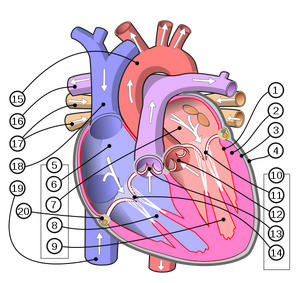
In addition to mechanical properties, some heart cells also exhibit a certain electrical activity and thus participate in triggering heart contractions - the so-called cardiac automation. We call the complex of these cells the cardiac conduction system [1] .
Nervous regulation ( sympathetic / parasympathetic ) also affects heart contractions , but it does not cause them, but only affects them (acceleration/deceleration). It is therefore not necessary for the activity of the heart itself, as much as for balancing the fluctuations caused by the load . [2]
Mechanical properties[edit | edit source]
The heart works as a pump consisting of four separate unidirectional pumps (two ventricles, two atria) and working as a synchronized double pump (small and large circulation). 90% of mechanical energy is used to maintain muscle tone, the remaining 10% is used for mechanical work, namely pressure-volume work (at high resistance pressure predominates, at high venous return volume predominance) and work indicating the acceleration by which it moves stroke volume mass versus ejection velocity.
Cardiac cycle[edit | edit source]
For more detailed information, see the Heart Revolution page .
The cardiac cycle describes the flow of blood between two heartbeats . The duration of one cardiac cycle is 0.75 s [3].
Progress[edit | edit source]
- Rapid ventricular filling phase, isometric phase
- The cardiac cycle begins with atrial systole , during which the ventricles are filled with blood (filling began before systole) [3].
Thanks to the mechanism of the valve plane, the resulting negative pressure also supports venous return. The systole itself then continues until the pressure in the atria (increased due to muscle contraction) is equal to the pressure in the ventricles. The alignment is manifested by the closing of the scalloped flaps as a result of the eddy currents in the niches on their "outer" side.
- Rapid expulsion phase, ejection phase
- The atria enter diastole, while the ventricles contract their muscles, which leads to a further increase in internal pressure. The latter causes the opening of the semilunar valves and expulsion of blood into the aorta and pulmonary artery. [3]. At the same time, thanks to the created negative pressure, the atria begin to fill with blood. [4]. Systole continues again until the pressures equalize. Then, thanks to the eddy currents in the niches on the "outer" side, the flaps close again. This phenomenon also induces vibratory movements on the arteries. However, approximately 50% of their full volume still remains in the ventricles - the so-called residual ventricular volume [3] .
- Isovolumic relaxation phase
- The ventricles enter diastole diastoly. However, due to the filling of the ventricles with blood in the previous phase, pressure differences between the atrium and the ventricle occur, which lead to the opening of the cuspid valves .
- Filling phase
- The ventricles begin to fill with blood. This phase is only interrupted by the contraction of the atrial muscle, which leads to the beginning of the isometric phase.
Heart sounds[edit | edit source]
During the cardiac cycle, we can listen to heart sounds. They are caused by valve closure . Systolic sounds can be heard during the closure of the leaflets in the isometric phase. Diastolic then during the closing of the semilunar valves in the ejection phase. (Listening to the types of heart sounds, see Sounds of the heart and lungs )
Flap opening and closing mechanism[edit | edit source]
The opening and closing of the heart valves is not controlled by the contraction of specialized muscles, but by the pressure of the flowing blood itself . The acting forces are therefore hydrodynamic .
The opening causes a pressure difference between the two compartments of the heart (atrium-ventricle or ventricle-vessel). As a result of this difference, the valves are dislodged towards the area with less pressure and thus allow a one-way blood transfusion. However, closing is more complicated. It occurs due to the eddy currents created in the recesses of the valve on the side where the blood spills over. After equalizing the pressures between the compartments, these currents "push" the valve back to its original location. The different surface of the lobed and crescent valves is also related to the mechanism of movement. This is caused by the disparity of pressures to which these valves must respond.
Defining the mechanical activity of the heart using physical quantities[edit | edit source]
The heart functions as a pump in the body and therefore performs a specific job. We can calculate this as the sum of kinetic work (spent to transmit a certain acceleration) and pressure-volume work (which moves a given volume of blood against the resistance in the vessels).
- Total work
- Pressure-volume work
The pressure-volume work of the heart acquires different physiological values in different compartments. This is mainly due to the difference in the function of the right and left parts of the heart. While the right contraction has the task of moving the blood "only" through a small circulation - i.e. to the lungs and back, the left one distributes already oxygenated blood throughout the body. It is therefore logical that the left side of the heart will show a much greater work done than the first part (beating frequencies are equal).
left ventricle | right ventricle | |
---|---|---|
Volume | 70 ml = 70×10−6 m3 | 70 ml = 70×10−6 m3 |
Pressure | 120 mmHg = 120 × 133 Pa | 15 mmHg = 15 × 133 Pa |
Pressure-volume work
Wp = p * V |
1,117 J | 0,140 J |
- Work causing acceleration
- m = stroke volume mass
- takes place in both chambers
- m = 74 g = 0,074 kg
- v = 0,5 m/s
- Wk = ½ × mv2 × 2 = 0,018 J [3]
- Effiency
The first law of thermodynamics states that no organ (or device) can use 100% of the energy consumed for its work. Part of this energy is "lost" for example by heat generation. Therefore, a quantity is introduced in physics that tells us what part of the energy is actually used for the required work. [5]
- Myocardial efficiency = 30%
- Whole heart efficiency = 10% (increases with exercise)
- Effective cardiac performance
Effective cardiac output is the output required to perform a certain work. It usually refers to the amount of blood ejected during one systole into the general circulation . Therefore, it is calculated by comparing pulse (heart rate) and ventricular residual volume .
- pulse (pulse) volume
- = amount of blood ejected by systole into the aorta
- depends on exertion and pathological conditions
- natural = 70-90 ml
- ventricular residual volume
- = amount of blood that remains in the ventricle after systole
- increases with pathological ventricular atrophy
- natural = around 50 ml
- Effective cardiac output values
- natural - between 0.55-0.67
- clearly reduced - less than 0.4
- EF = (eDV - eSV)/eDV
- EF = effective power
- eDV = ventricular volume at end diastole
- eSV = ventricular volume at the end of systole
- eDV - eSV = ventricular residual volume
- Minute volume
- = amount of blood expelled in one minute
- values are highly variable
- on average – 5000–9000 ml/min
- V60=Vp*P60
- V60 = minute volume
- Vp = stroke volume
- P60 = number of pulses per minute
Electrical properties[edit | edit source]
More information in the article Cardiac conduction system
The heart has its own automation, which means that it creates its own stimuli for the heart muscle to contract. The transmission of the heart impulse is mediated by heart cells, which can be divided into 3 types according to their form and function: nodal myocytes' (considered as the source of charge), transient type myocytes (propagating the signal between nodal myocytes and cells of the Purkinje fiber type) and myocytes of the Purkinje fiber type (responsible for the rapid conduction of the impulse to the cells of the myocardium).
Heart activity[edit | edit source]
Mechanical and electrical activity are not strictly separated. They take place at the same time and the mechanical one is electrically controlled.
- Simplified course of heart contraction:
- sinus node irritation
- atrial systole -> replenishment of ventricles with blood (they begin to fill even before atrial systole)
- transmission of irritation from the right atrium to the ventricles
- ventricular systole -> ejection of blood into the artery
- diastole and filling of the heart with blood [2]
Vessels[edit | edit source]
Vessels are the distribution part of a closed circuit. With their elasticity, extensibility and active contractility, they participate in keeping the blood moving. Vessels are divided into arteries, veins and capillaries, each of which has a different meaning in conducting blood and together form the blood channel system. In arteries blood flows at a high rate and a pressure wave that is palpable as a pulse spreads through them. In veins, blood flows more slowly and under lower pressure than in arteries, therefore the flow of blood in the veins must be assisted by other mechanisms, mainly the valve system and the muscular work of the lower limbs (sources 2, 3). Capillaries have easily permeable walls, and blood flows through them at a constant speed without pressure fluctuations, therefore there is an exchange of substances with the surrounding tissues.
We can divide them into three circulatory systems:
- coronary circulation ensuring blood supply to the heart
- pulmonary circulation ensuring blood saturation with oxygen [2]
- great circulation ensuring the distribution of oxygenated blood to the body
Flow in vessels[edit | edit source]
Considering the individuality of each individual, it is difficult to accurately characterize the influences acting on individual events, that is why we say that the laws of physics biomechanics of liquids apply here approximately. The Bernoulli's law and Hagen-Poiseuille's law are mainly applied when blood flows in the vessels.
Bernoulli's Law[edit | edit source]
Bernoulli's law is expressed by:
,
where v is the flow velocity, P is the pressure, ρ is the density, g is the gravitational acceleration.
At a location with a larger cross-section, the flowing fluid has a higher pressure but a lower velocity, while at a location with a smaller cross-section it has a lower pressure but higher speed. The pressure of the flowing liquid therefore decreases with increasing velocity.
Hagen-Poiseuille law[edit | edit source]
Hagen-Poiseuille law is given by the following relation:
where Q is the volume flow, ΔP is the pressure difference at the beginning and end of the tube, η is the dynamic viscosity of the liquid, R is the radius of the tube, L is the length of the tube. The dependence on the fourth power of the radius in Hagen-Poiseuille's law causes that, for example, a decrease in the radius of an arteriole due to the contraction of the smooth muscles in its walls by 19% will cause a reduction in blood flow to approximately half. [3]
Laminar and turbulent flow[edit | edit source]
Under normal conditions, the flow of blood in the vessels is laminar, whereby the layer of blood close to the wall of the vessel hardly moves and towards the center of the vessel the speed of the flow increases. Laminar flow, however, is maintained only up to a certain critical velocity, and above it the flow becomes 'turbulent. This boundary value is determined using Reynolds numbers. The flow changes at values of this number around 2000, at lower values it is laminar, at higher values it is turbulent flow [3]. The Reynolds number is given by:
where R is the Reynolds number, ρ is the liquid density, d is the tube diameter, v is the flow velocity, η is the liquid viscosity
Elastance and vascular compliance[edit | edit source]
The time course of ventricular stiffness during the cardiac cycle is expressed by elastance ( E) , which is defined as:
where p is pressure and V is volume.
The inverse value is given as compliance C (compliance) of vessels, here given by the relation:
where p is pressure again and V is volume.
Blood pressure[edit | edit source]
Blood pressure is the pressure exerted by flowing blood on the vessel wall . The value of blood pressure is different in different parts of the bloodstream, the highest is in the large arteries , it decreases towards the periphery, and the lowest is in the venous system . The term blood pressure most often refers to arterial blood pressure. Arterial blood pressure changes during the cardiac cycle , the highest is in the ejection phase = systolic pressure , the lowest during the filling phase = diastolic pressure . The difference between systolic and diastolic pressure is the pressure (pulse) amplitude. The blood pressure value consists of two numbers separated by a slash, the higher number is the systolic pressure value, the lower number is the diastolic pressure (expressed most often in mmHg, less often in kPa). E.g. a pressure value of 120/80 mmHg means that the systolic pressure is 120 mmHg and the diastolic pressure is 80 mmHg. For a healthy adult, the systolic pressure value should not exceed 130 mmHg and the diastolic pressure value should not exceed 90 mmHg. Low blood pressure is referred to as hypotension , high blood pressure is hypertension [6].
Systolic pressure[edit | edit source]
Systolic pressure ( ST ) is the pressure in the large arteries at the peak of the ejection phase. It is influenced by the degree of myocardial contractility and the volume of ejected blood. In a healthy adult, it should not exceed 130 mmHg (17.3 kPa) [3].
Diastolic pressure[edit | edit source]
Diastolic pressure ( DT ) is the lowest blood pressure in the large arteries. It occurs at the end of the isovolemic phase before the aortic valve closes . Its value is most influenced by the degree of elasticity of the walls of the large arteries . Even during diastole, the blood pressure in the vessels never drops to zero (unlike the heart chambers ). Blood circulation during diastole is maintained by blood that has accumulated in dilated vessels. In a healthy adult, the value of diastolic pressure should not exceed 90 mmHg (11.3 kPa) [3].
Medium pressure[edit | edit source]
Mean arterial pressure ( SAT ) is the average value or, more precisely, the mean value of blood pressure during one cardiac cycle . The value of mean arterial pressure cannot be calculated as the arithmetic mean of the value of systolic (ST) and diastolic (DT) pressure, because the course of blood pressure depending on time is quite complex. Above all , diastole lasts approximately twice as long as systole at a resting heart rate . For this reason, the SAT value is closer to the diastolic pressure value. At physiological blood pressure values (120/80 mmHg), the mean arterial pressure value is 93.3 mmHg.
For an accurate calculation, it would be necessary to know the detailed course of arterial pressure values during the entire cardiac cycle. This condition is partially fulfilled only during invasive measurement, therefore, when estimating the mean arterial pressure, relations using the fact that SAT significantly depends on the value of systolic and diastolic pressure are used:
respectively
respectively
- [9]
SAT= mean arterial pressure, DT= diastolic pressure, ST= systolic pressure
Pressure amplitude[edit | edit source]
Pressure amplitude ("TA") or pulse pressure is the difference between systolic and diastolic pressure. At physiological blood pressure values (120/80 mmHg), the pressure amplitude is equal to 40 mmHg. The pressure amplitude is greatest in the large arteries, decreasing towards the periphery. The size of the pressure amplitude is mainly determined by the systolic volume (an increase in the systolic volume leads to an increase in the pressure amplitude) and the compliance of the vessels (greater compliance of the vessels reduces the pressure amplitude).
- [7].
TA=pressure amplitude, ST=systolic pressure, DT=diastolic pressure
Orthostasis[edit | edit source]
Orthostasis is the upright position of the body. In this position, they have other influences on the pressure in the blood vessels than the activity of the heart. Towards the periphery of the body, the pressure amplitude decreases and the influence of factors other than heart activity increases, primarily hydrostatic pressure (especially when standing upright). For 1 cm of height "from heart to heel" there is an increase in pressure of 1 mmHg. On the other hand, in the head region, the venous pressure can be negative due to gravity in an upright position. Gravity acts equally on veins and arteries , so the difference between them is negligible. Since the vascular system is closed, we will measure pressure values lower than we would measure in a system of tubes that is open at the top. It lies about 5–10 cm below the diaphragmhydrostatically indifferent plane , here the blood pressure does not change when the position changes. Subatmospheric pressure acts against the collapse of the chest veins. [3]
Links[edit | edit source]
[edit | edit source]
External links[edit | edit source]
- Article Cardiac cycle on the Czech Wikipedia
- Presentation on the CTU website
Reference[edit | edit source]
- ↑ a b
- ↑ a b c NOVOTNÝ, Ivan – HRUŠKA, Michal. Human biology : pro gymnázia. 3. edition. Prague : Fortuna, 2003. ISBN 8071688193.
- ↑ LEJSEK, Jan. Poznámky z fyziologie [online]. [cit. 2014-05-19]. <http://stary.lf2.cuni.cz/projekty/mua/3y1.htm>.
- ↑ TARÁBEK, Pavol – ČERVINKOVÁ, Petra, et al. Graduate! from physics. 2. edition. Brno : Didaktis, c2006. ISBN 8073580586.
- ↑ ŠVÍGLEROVÁ, Jitka. Střední arteriální tlak [online]. [cit. 27.11.2013]. <https://web.archive.org/web/20160306065550/http://wiki.lfp-studium.cz/index.php/St%C5%99edn%C3%AD_arteri%C3%A1ln%C3%AD_tlak>.
- ↑ ŠVÍGLEROVÁ, Jitka. Tlaková amplituda [online]. [cit. 27.11.2013]. <https://web.archive.org/web/20161225092053/http://wiki.lfp-studium.cz/index.php/Tlakov%C3%A1_amplituda/>.
Literature[edit | edit source]
- ČIHÁK, Radomír. Anatomie 3. 2. vydání. Praha : Grada Publishing, 2004. 692 s. ISBN 978-80-247-1132-4.
- NOVOTNÝ, Ivan a Michal HRUŠKA. Biologie člověka : pro gymnázia. 3. vydání. Praha : Fortuna, 2003. ISBN 8071688193.
- NAVRÁTIL, Leoš a Jozef ROSINA, et al. Medicínská biofyzika. 1. vydání. Praha : Grada, 2005. 524 s. ISBN 80-247-1152-4.
- LEJSEK, Jan. Poznámky z fyziologie [online]. [cit. 2014-05-19]. <http://stary.lf2.cuni.cz/projekty/mua/3y1.htm>.
- Zvuky srdce a plic [online]. Praha, 2015 [cit. 2015-11-24]. Dostupné z: http://solutions.3mcesko.cz/wps/portal/3M/cs_CZ/Littmann/stethoscope/education/heart-lung-sounds/
- TARÁBEK, Pavol a Petra ČERVINKOVÁ, et al. Odmaturuj! z fyziky. 2. vydání. Brno : Didaktis, c2006. ISBN 8073580586.
- ŠVÍGLEROVÁ, Jitka. Střední arteriální tlak [online]. [cit. 27.11.2013]. <https://web.archive.org/web/20160306065550/http://wiki.lfp-studium.cz/index.php/St%C5%99edn%C3%AD_arteri%C3%A1ln%C3%AD_tlak>.
- ŠVÍGLEROVÁ, Jitka. Tlaková amplituda [online]. [cit. 27.11.2013]. <https://web.archive.org/web/20161225092053/http://wiki.lfp-studium.cz/index.php/Tlakov%C3%A1_amplituda/>